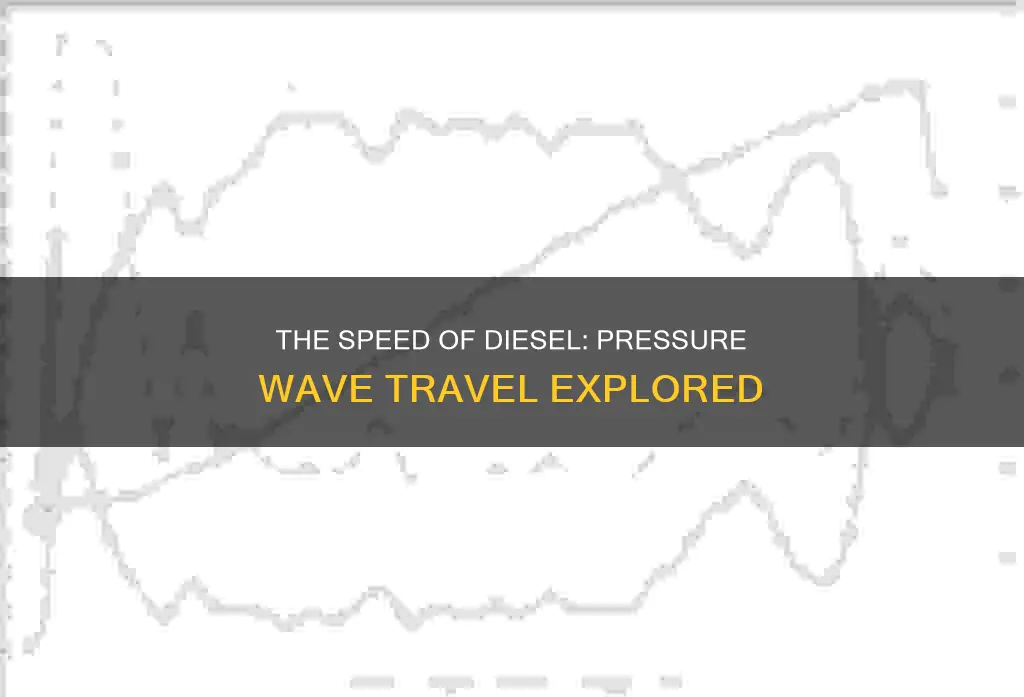
The latest generation of high-pressure common rail equipment now provides diesel engines with the possibility of applying as many as eight separate injection pulses within the engine cycle. This helps to reduce emissions and smoothen combustion. However, when using multiple injections with a very short interval between injection pulses, the effect of the pressure wave in the fuel pipe on the subsequent fuel injections must be considered. Due to the fast opening and closing of the injector needle, injection pressure waves are generated in the duct of the common rail, injectors, and the connecting high-pressure pipe. These pressure waves then push up or down the subsequent injection amount significantly, making the calibration of the subsequent injection fuel amount very difficult. Therefore, it is essential to have a full understanding of this phenomenon.
Characteristics | Values |
---|---|
Pressure wave in common rail injection system | Prail - pressure in rail; V - flow velocity; P - pressure in fuel pipe |
Test engine and measurement equipment | 2.2-L four-cylinder four-valve turbocharged DI diesel engine |
Common rail fuel injection system | Delphi HPCR fuel injection; 1600-bar maximum fuel injection pressure; 0.12 mm orifice diameter of the nozzles; 6 holes in each injector |
What You'll Learn
- Pressure waves in the common rail can cause oscillations in fuel injection rate
- Pilot injection timing affects the stability of main injection and combustion
- Injector design can minimise pressure waves in high-pressure lines
- Pressure waves in the common rail can be controlled by optimising rail orifices
- Algorithms can be used to predict and adjust for errors caused by pressure waves
Pressure waves in the common rail can cause oscillations in fuel injection rate
The rail pressure can be controlled to a value that suits the engine's operating conditions. In some cases, rail pressures can reach 300 MPa. The common rail system is prone to effects related to wave dynamics in the rail and fuel lines. Waves generated by sudden pressure changes in one part of the system may reflect at rigid terminations and return to their origin, causing unwanted consequences such as reduced injection pressure and variations in injection quantity.
The occurrence of high-amplitude, low-frequency pressure waves during the injection event is one of the most significant challenges in reducing the dwell time between multiple injections. A significant attenuation of pressure oscillations can be achieved by selecting the appropriate dimensions for the injector inlet pipe. The energy stored in pressure waves induced by injection events with the same injection duration and rail pressure remains almost constant when the geometrical parameters of the injector supply pipes are modified.
The frequency of the pressure waves is strictly related to the geometric features of the high-pressure circuit. Therefore, the focus is on designing the circuit to maximise the frequency of the waves. Physical modelling systematically shows that this frequency increases with the injector inlet pipe aspect ratio (the ratio of length to the internal diameter). Modulating pressure-wave oscillations in this way is considered an active damping strategy.
Alternatively, the introduction of orifices at the rail-to-pipe connections or inside the injector can be used. This is considered a passive damping strategy. For a particular injection duration and rail pressure, an orifice will generally decrease the injected fuel quantity when compared to a hydraulic layout without an orifice. The relative reduction is variable but is typically less than 10%. An orifice will also reduce injection system hydraulic efficiency.
A relatively large volume accumulator has traditionally been considered fundamental to dampening the pressure fluctuations caused by the fuel pulses delivered by the pump and the fuel-injection cycles in common rail systems. However, studies with a fuel injection system for light vehicles have shown that the progressive reduction in the accumulator volume from 20 to 3 cm3 has no impact on the amplitude of these pressure fluctuations and little negative impact on injector performance. The high-pressure control capability of the system in these studies resulted from the synergic action of both the system's high-pressure hydraulic capacitance and the pressure control device.
The fuel injection rate and fuel injection duration are different with different fuel injection pulse widths, which results in different cycle fuel injection volumes. The change in injection pulse width has a different influence on pressure fluctuation characteristics in the system when the fuel is injected. In addition, the cycle fuel injection volume is different even if the injection pulse width is the same when the high-pressure common rail fuel injection system is under different common rail pressures.
The dynamic pressure wave of the system shows different fluctuation characteristics under different injection pulse widths and common rail pressures when the size of the high-pressure pipe is constant. It either fluctuates at the main crest frequency or shows the characteristics of multi-frequency fluctuation. While the dynamic pressure wave of the system shows low-frequency fluctuation under a low common rail pressure at the same injection pulse width.
The average injector inlet pressure varies with different common rail pressure and injection pulse width. The difference between the average injector inlet pressure and the set common rail pressure not only reflects the decreased amplitude of injection pressure in the fuel injection process but also reflects the average amplitude of pressure fluctuation in the system after fuel injection. Therefore, the average pressure drop is defined in this chapter as the difference between the set common rail pressure and the average injector inlet pressure which is located at the moment of injector solenoid valve energised time coordinates from 0 to 10 ms. The average pressure drop increases linearly with the increase of common rail pressure from 40 MPa to 160 MPa when the size of the high-pressure fuel pipe and injection pulse width is constant. The larger the injection pulse width, the faster the average pressure drop increase rate.
The first trough of the pressure wave at the injector inlet is the minimum pressure of the system after the fuel injection when the nozzle hole is opened, which reflects the maximum pressure drop after stable fuel injection. The first crest of the injector inlet pressure is the maximum compression wave returned in the system when the injection pulse width is large. It reflects the pressure fluctuation amplitude of hydraulic resonance when the injection pulse width is small. Therefore, the first trough and the first crest of the injector inlet pressure are the characteristic parameters reflecting the dynamic pressure wave characteristics of the system.
Understanding Fuel Pressure in the Chevy Cruze Diesel
You may want to see also
Pilot injection timing affects the stability of main injection and combustion
The pilot injection timing has a significant impact on the stability of the main injection and combustion process in diesel engines. By optimising the timing, it is possible to improve combustion stability, reduce emissions, and enhance engine performance. Here are four to six paragraphs explaining the effects of pilot injection timing on the stability of main injection and combustion:
Pilot injection is a technique used in diesel engines to reduce ignition delay and lower the rate of pressure rise at the start of injection. The timing of this pre-injection of fuel can have a significant impact on the stability of the main injection and combustion process. Advancing the pilot injection timing can lead to better overall combustion and improve engine performance. This is because advancing the timing allows for better spray jet penetration and development, resulting in a more complete and efficient combustion process.
The pilot injection timing also affects the combustion pressure and heat release rate. By optimising the timing, the combustion pressure can be reduced, which, in turn, lowers the rate of heat release. This not only improves combustion stability but also helps to reduce engine noise and NOx emissions. The pilot injection acts as a pre-injection, reducing the ignition delay and preventing rapid increases in temperature and pressure. This, in turn, leads to lower NOx emissions and improved fuel efficiency.
Additionally, the pilot injection timing plays a crucial role in dual-fuel engines, where it can be used to improve combustion and reduce emissions. In dual-fuel engines, a small amount of diesel is injected first, followed by the injection of the gaseous fuel. Advancing the pilot injection timing in these engines can improve the spray jet penetration and enhance the development of the diesel spray. This, in turn, improves the overall combustion process and reduces the formation of high-pressure and high-temperature conditions that favour NOx production.
Furthermore, the pilot injection timing can be adjusted to reduce fuel consumption. By optimising the timing, the ignition delay period can be decreased, resulting in lower temperatures and pressures during the preliminary phase of combustion. This leads to a slight reduction in fuel consumption while also minimising particulate matter emissions. The reduction in ignition delay also helps to compensate for any shortcomings in engine performance.
The pilot injection strategy, which includes the timing, size, spacing, and pressure, is crucial for achieving stable engine operation and maintaining performance without power loss. By optimising the pilot injection timing, it is possible to enhance combustion and reduce undesirable emissions, such as NOx and particulate matter. This makes the pilot injection timing a vital parameter in the overall engine performance and emissions control strategy for diesel engines.
Fuel Pressure Maintenance for the 1993 Chevy C1500
You may want to see also
Injector design can minimise pressure waves in high-pressure lines
Injector design plays a crucial role in minimising pressure waves in high-pressure lines of diesel fuel injection systems. The dimensions of the injector supply pipe and the rail volume are critical parameters that significantly impact the injection system's dynamic performance. By optimising these components, manufacturers can reduce complications arising from line dynamics, such as erratic injection behaviour at the nozzle.
One strategy to minimise pressure waves is to keep the total line length as short as possible. In some cases, however, even the shortest line may be too long for effective injection. This issue can be addressed by using unit pump systems, where each unit pump is installed close to the cylinder it serves, ensuring shorter injection lines.
Another approach is to utilise a distributor pump system, where a central pumping element produces high-pressure fuel that is then diverted to the appropriate injector and cylinder. This reduces the number of costly high-precision pumping elements required for multi-cylinder engines.
Additionally, the introduction of orifices at the rail to pipe connections or inside the injector can be used as a passive damping strategy to minimise pressure waves. While this may result in a slight reduction in the injected fuel quantity, it helps to control pressure oscillations.
Furthermore, modern common rail systems incorporate additional accumulator volume in the injector to better control the pressure at the injector nozzle. This design feature aids in reducing the amplitude of pressure waves during the injection event.
Overall, injector design plays a pivotal role in mitigating pressure waves in high-pressure lines, ensuring the efficient and controlled delivery of diesel fuel in injection systems.
Fuel Pressure Monitoring: Safety, Performance, and Engine Health
You may want to see also
Pressure waves in the common rail can be controlled by optimising rail orifices
The rail pressure can be controlled to a value that depends on the needs of any particular engine operating condition. Rail pressures can reach 300 MPa. However, common rail systems are prone to effects related to wave dynamics in the rail and in fuel lines. Waves generated by sudden changes in pressure in one part of the system may become reflected at rigid terminations and return to their origins, causing reduced injection pressure and variations in injection quantity.
To better control the pressure at the injector nozzle, some common rail injectors include an additional accumulator volume in the injector. The occurrence of high amplitude/low-frequency pressure waves during the injection event is one of the most important challenges in reducing the dwell time between multiple injections. Reducing the amplitude of these oscillations is an important objective of fuel injection system designers.
The energy stored in pressure waves induced by injection events remains almost constant when the geometrical parameters of the injector supply pipes are modified. However, the energy stored in a sinusoidal pressure-wave train increases with the square of both its amplitude and frequency. Therefore, hydraulic layout modifications leading to increased pressure-oscillation amplitudes should yield reduced frequencies and vice versa.
The frequency of the pressure waves is strictly related to the geometric features of the high-pressure circuit. Therefore, the focus is on designing the circuit to maximise the frequency of the waves. Physical modelling shows that this frequency increases with the injector inlet pipe aspect ratio (the ratio of the length to the internal diameter). Modulating pressure-wave oscillations in this way is considered an active damping strategy.
Alternatively, the introduction of orifices at the rail-to-pipe connections or inside the injector can be used. This is considered a passive damping strategy. For a particular injection duration and rail pressure, an orifice will generally decrease the injected fuel quantity when compared to a hydraulic layout without an orifice. The relative reduction is variable but is typically less than 10%. An orifice will also reduce injection system hydraulic efficiency.
A relatively large volume accumulator has traditionally been considered fundamental to dampen the pressure fluctuations caused by the fuel pulses delivered by the pump and the fuel injection cycles in common rail systems. However, studies have shown that the progressive reduction in the accumulator volume from 20 to 3 cm3 has no impact on the amplitude of these pressure fluctuations and little negative impact on injector performance. The high-pressure control capability of the system in these studies resulted from the synergic action of both the system's high-pressure hydraulic capacitance and the pressure control device. Although the duty cycle of either the pressure control valve or the fuel metering valve at the pump inlet depended on the rail size, the high-pressure control system was capable of keeping the pressure level adequately close to the nominal value for the range of accumulator volumes studied.
This finding has been applied to the design of newer-generation common rail systems for passenger cars, which use smaller rail volumes than in the past. It also opens the door to the possibility of removing the rail entirely from the high-pressure circuit. Such a system concept, referred to as Common Feeding, uses a small hydraulic accumulator volume integrated into the pump, which is then connected directly to the injector feed lines. The resulting injection system has low hydraulic inertia, giving rise to a fast dynamic response during transients and reduced production costs. Furthermore, this system matches the requirements of easy installation on the engine.
While there are some notable differences in the system behaviour compared to a common rail, these can be accommodated in the design and calibration. Some of the differences include a larger pressure drop and variations in the frequency of free pressure waves in the high-pressure circuit. For pilot-main injections, orifices are required at the accumulator outlets to dampen the pressure waves triggered by the pilot injection and minimise its effect on the main injection quantity.
Selecting the Right Fuel Injection Pressure Tester Kit
You may want to see also
Algorithms can be used to predict and adjust for errors caused by pressure waves
The accuracy of PWV estimates deteriorates for higher PWV values, and this is mainly due to the number of subjects with high PWV being far less than those with lower PWV. As machine learning algorithms are data-driven, the scarceness of subjects with high PWV makes estimation for higher PWV values more challenging. The accuracy of the PWV estimates could be improved by training the algorithms with a training database containing more subjects with high PWV values.
In addition, the prediction errors of the final model were within the range of about 9 to 12 mmHg that can occur with a gold standard mercury sphygmomanometer. This suggests that the model would be efficient and feasible for predicting blood pressure.
Replacing the Fuel Tank Pressure Sensor in a W204: Step-by-Step Guide
You may want to see also
Frequently asked questions
A pressure wave is a phenomenon that occurs in a common rail fuel injection system when the valve in the injector is closed quickly, creating a "water hammer" in the injector pipe. This pressure wave travels toward the main rail, causing an imbalance in pressure that pushes the fuel back and forth, resulting in an oscillation that is eventually damped.
The pressure wave in the common rail can significantly influence subsequent injections, making the calibration of the fuel amount very difficult. The effect is more pronounced when there is a short interval between injections, and it can cause fluctuations in the actual fuel amount delivered.
The oscillation frequency of the pressure wave relative to time remains the same for different engine speeds, but the oscillation frequency based on crank angle changes with engine speed.
To counter the effects of the pressure wave, fuel injection equipment manufacturers use various approaches, including optimizing rail orifices to dampen the wave and using algorithms to predict and adjust for the error. Some injectors are also specially designed to minimize the wave in the high-pressure lines.